Hybrid Nd:YAG Laser/Gas Metal Arc Welding for New Land Pipelines
G S Booth[1], D S Howse[1], A C Woloszyn[1] and R D Howard[2]
[1]TWI
[2]BP Exploration
Paper presented at Pipeline Construction Technology Conference, Wollongong, NSW, Australia, 4 & 5 March 2002
Abstract:
World demand for natural gas has generated the need for many new land transmission pipelines to be installed in the next decade or so. Although mechanised gas metal arc welding is well developed, there are opportunities for cost savings by using alternative welding processes.
Hybrid Nd:YAG laser - gas metal arc welding enables fibre optic delivery of the laser energy to a robotic welding head to be combined with the addition of extra energy and a consumable to produce good quality, deep penetration weldsin a single pass.
The present paper describes initial welding trials and procedure development to optimise the laser and gas metal arc welding parameters for making joints in pipeline steel. Satisfactory joint quality and hardness values were obtained, and it is intended to develop the process to prototype field trials.
Keywords
Nd:YAG laser, Penetration, GMAW, Porosity, Hybrid laser-arc, Hardness, Pipeline steel
1. Introduction
1.1 Background
World demand for natural gas is expected to be satisfied for the next decade or so by the exploitation of current known gas reserves. Many of these reserves, however, are situated in remote locations and there is a need for land transmission pipelines to transport this 'stranded gas' from its source to where it is in demand.
As there are large costs associated with laying new pipelines, particularly in remote and possibly hostile environments, very significant gains can be made by identifying and implementing cost savings.[1] A wide range of opportunities for cost savings has been targeted, including pipeline concept, design, specification of materials, procurement of materials and services, construction and operation. Clearly pipeline welding has a bearing on costs, and two aspects are particularly important. Firstly, the speed of welding largely determines the pace of construction. Secondly, the number of people employed on site as welders, fitters and associated logistics support staff contributes significantly to the total cost of welding.
The present paper briefly reviews candidate welding processes and technologies that offer opportunities for cost savings. One attractive candidate process, hybrid laser/arc welding, is then considered in more detail and initial experimental results are presented.
1.2 Candidate Welding Processes
Taking as a base case the construction of a 500km gas export pipeline in 48in diameter, 0.688in (17.5mm) wall thickness, API 5L-X70 pipe in North African desert conditions, TWI and BP staff recently carried out a brainstorming exercise to assess candidate welding processes.[2] Mechanised gas metal arc welding (GMAW) was taken as the benchmark process and four other processes were selected for further evaluation:
- fully automated GMAW
- friction welding
- reduced pressure electron beam welding
- laser welding
Mechanised GMAW systems are well developed, have a proven track record for land lay, and may be considered state of the art for high productivity welding of large diameter onshore pipelines. A disadvantage, however, is that multiple welding stations and weld passes are required to complete each joint. Hence, it remains relatively labour intensive.
In a fully automated GMAW system, the operator simply positions the machine at the start of the joint and monitors the weld, and has no influence at all over the welding process. Although one fully automatic process is used offshore, the conceptual design for onshore pipe lay presents additional challenges because welding is carried out at a series of stations rather than on a barge. However, dual torch technology is one way forward and some cost saving gains are achievable.
Friction welding is a solid phase forge welding process where one component is rotated against another and frictional heating is used to make a weld under the application of an axial force. Given the circular shape of pipelines, friction welding is particularly suitable and there are three possible approaches:
- rotation of one pipe in contact with a stationary pipe
- rotation of a short piece of pipe between two stationary pipes (a 'pup' piece)
- rotation of a bevelled insert ring between two stationary pipes (radial friction welding)
Although each of these processes is theoretically feasible, significant engineering challenges, such as rotating a 12m length of pipe and applying an axial force, would have to be overcome. To date, relatively limited commercial system development has been undertaken, with the notable exception of radial friction welding for offshore pipe lay of smaller diameter pipelines, typically up to 324mm diameter. [2]
Electron beam welding is a single pass fusion welding process which uses a focussed high energy beam of electrons to melt material and make a weld. In the 1960s and 1970s, early development of pipeline welding took place but was limited by the need for high vacuum operation. In the 1990s, however, TWI developed a system that can weld in the pressure range 0.1 to 10 mbar and showed that good weld quality could be achieved without the need for sophisticated high vacuum engineering. This technology is particularly suited to offshore J-lay applications where welding is in the 2G position (pipe vertical/weld horizontal).[3] Significant development work is required to apply reduced pressure electron beam welding in the 5G position (pipe horizontal/welding position varying) for land lay.
Laser welding uses a coherent beam of light of a single wavelength to produce a deep penetration fusion weld by focussing the beam to a small spot size. This enables high joining rates to be achieved with low workpiece distortion. The process is fully automated and eliminates manual operator skill. Additionally, the Nd:YAG laser produces light with a wavelength that can be transmitted by an optical fibre, which enables the laser welding head to be installed on a conventional robot.
A summary [4] of the key processing, production and economic parameters associated with each of the five welding processes is presented in Table 1. Although laser welding has relatively high capital costs, manning levels are low and productivity levels are similar to those for the benchmark process - mechanised GMAW.
Table 1 Summary of key parameters [4]
Gas export pipeline, 500km long, 48 in diameter, 0.688 in (17.5mm) wall thickness.
Process | Welds per Day (a) | Manning Level | Capital Costs (b) | Labour and Consumable Costs per Weld (c) | Status of Development |
Mechanised Gas Metal Arc Welding |
50 to 150 |
50 |
$150,000 |
$160 to 270 |
Fully developed, incremental improvements possible |
Automated Gas Metal Arc Welding |
40 to 100 |
15 |
£2-3M |
$140 to 180 |
Not developed |
Friction Welding
Conventional Rotating Pup Pieces Radial
|
|
No data available No data available Not suitable |
|
|
|
Electron Beam Welding |
60 |
2-4 |
$4-8M |
$20-$40 |
Near to industrial exploitation for J lay applications. Needs development for land pipelines welded in the 5G position |
Laser Welding
CO 2 Source Nd:YAG Source
|
60 - 100 60 - 100 |
8 - 10 8 - 10 |
$4 - 8M $4 - 8M |
$30 - $60 $30 - $60 |
Prototype equipment available R & D stage |
(a) 10 Hour day (b) Mobilisation costs for GMAW (c) Labour rate assumed to be $45/hour |
1.3 Laser Welding for Land Lay Pipelines
Due to the narrow, deep penetration weld produced, laser welding offers several advantages:
- high joining rates
- low consumable costs
- a reproducible, machine tool welding process
- low manning levels
- low degrees of distortion, leading to greater precision in assembly and reduced rectification
Two main types of laser are available industrially for welding thick section steel - CO2 gas lasers and Nd:YAG solid state lasers. CO2 lasers generate light in the infrared regime at a wavelength of 10.6µm and are available at power levels up to about 45kW. The light is transmitted from the laser to the workpiece by a system of mirrors, before being focussed to a small spot for welding. This type of laser is now being used in four European shipyards for different aspects of ship fabrication, where the reduced distortion enables the costs associated with rework to be dramatically reduced. This type of laser has been considered and evaluated forS-lay of offshore pipelines. [5]
Nd:YAG lasers, in contrast, generate light at a wavelength of 1.06µm, which is also in the infrared regime, but can be transmitted by a fibre-optic cable. This is a major advantage, as it enables the complex set of mirrors usedfor the beam path of a CO2 laser to be replaced by a simple fibre and welding head that can be mounted on a welding robot - Figure 1.
Fig. 1. Nd:YAG welding head position in robot arm, showing fibre delivery capability
Advances in laser technology have led to rapid increases in the power available in Nd:YAG lasers and 4kW Nd:YAG lasers are now extensively used in production. A beam combining unit at TWI enables three lasers to be coupled together to achieve processing capability with up to 10kW.
An initial laboratory trial at TWI to demonstrate Nd:YAG laser welding of a land pipeline is illustrated in Figure 2. These trials were followed by a research programme currently in progress involving BP and CRC-Evans, in which an internal GMAW root run is made using conventional techniques, a laser weld is then made from the outside and a final GMAW capping pass is deposited. A typical weld cross section is shown in Figure 3. This approach enables the number of welding stations to be reduced to 3 or 4, whilst maintaining the overall pace of welding to that of the benchmark GMAW process.
Fig. 2. Initial laboratory trials using a Nd:YAG laser for pipeline welding
Fig. 3. Section through 16mm thick pipe wall, showing internal GMAW root run, laser fill and GMAW capping pass (mm scale shown)
Laser welding alone, however, is a demanding process and very tight control of a number of parameters such as fit-up and steel composition is required in order to produce welds of satisfactory quality and performance. Recent attention, therefore, has concentrated on hybrid laser-arc welding, which offers an enhanced range of applicability.
1.4 Hybrid Laser-Arc Welding
Hybrid laser-arc welding refers to the coupling of the two welding processes into a single process area or weld pool, as depicted in Figure 4. The options available include combining either CO2 orNd:YAG laser welding with an arc welding process such as gas metal arc welding, gas tungsten arc welding or plasma arc welding.
Fig. 4. Hybrid laser-arc welding
Compared with the use of laser power alone, hybrid laser-arc welding offers the following benefits:
- increased travel speed or penetration
- improved tolerance to fit-up gap
- ability to add filler material to improve weld metal microstructure, joint quality and properties
- potentially improved energy coupling
- increased heat input, and reduced hardness
There are, however, some drawbacks which include increased process complexity ('more things to go wrong'); additional welding parameters to be defined; and the requirement to define the process parameters anew as these cannot simply be determined from the two separate processes.
Arguably, the most suitable approach for land pipeline welding is to combine a Nd:YAG laser with GMAW because this arrangement offers the opportunity to add filler material combined with fibre delivered laser power. Initial experimental results to develop suitable welding parameters are now presented.
2. Experimental programme
2.1 Materials
Steel pipe, complying with the requirements of API 5L X-60 and of dimensions 762 mm outside diameter, 15.9 mm wall thickness, was used for this work. The chemical composition of the steel is given in Table 2.
Table 2 Steel Composition (wt%)
C | S | P | Si | Mn | Ni | Cr | Mo | V | Cu | Nb |
0.11 |
0.004 |
0.021 |
0.33 |
1.42 |
0.023 |
0.018 |
0.003 |
0.002 |
0.023 |
0.040 |
|
Ti | Al | B | Sn | Co | As | Ca | O | N | CE | |
0.003 |
0.045 |
0.0003 |
<0.004 |
<0.004 |
<0.004 |
0.0001 |
0.0008 |
0.0032 |
0.35 |
|
2.2 Equipment
A welding system, which integrated the Nd:YAG laser welding head and a GMA welding torch was designed and built. The fixture allowed accurate and repeatable control of the relative positions and orientations of the two heat sources and was mounted on a Kawasaki Js-30 robot, as shown in Figure 5.
Fig. 5. Laboratory demonstration of hybrid Nd:YAG laser/GMA welding for pipelines
The laser energy was provided by two Nd:YAG lasers each producing infrared light of wavelength 1.064 µm. The two lasers were a multiwave AutoTM built by GSI Lumonics and a Trumpf HL 3006 D. The outputs from the two laser sources were fed into a Beam Combining Unit which enabled the combined laser power to be output through a single fibre optic of 1 mm diameter to the welding head which comprised recollimating and focusing lenses configured to produce a spot size of 1.4 mm diameter at a lens to workpiece stand-off distance of 220 mm. The total laser power at the workpiece was 5.2kW.
The GMA welding system was a Lincoln Electric Powerwave 455, capable of delivering up to 400A welding current. A synergic pulsed welding programme was used.
2.3 Welding Procedure Development
2.3.1 Initial trials
To establish initial conditions, bead on plate welds in the flat position were produced, with the weld progressing along a flat surface parallel to the pipe axis. The following parameters were used:
- GMA process leading
- Zero arc-laser separation distance
- 0.6 m/min travel speed
- GMA torch at 60° to the horizontal
- 3 kW (130A/23V) GMA arc power
- 1 mm diameter Thyssen K-Nova 2% Ni solid filler wire
- 20 mm electrode extension
- Ar- 18% CO 2 -2% O 2 shielding gas
- 5.2 kW laser power at workpiece
- 0 and 3 mm sub surface laser focus position
Welds were produced using laser welding alone, GMAW alone and hybrid laser-GMA welding.
2.3.2 Process variables
Based on TWI experience and a review of literature, it was considered that the four most important variables were GMA current, arc-laser separation distance, lead process and travel speed. A full-factorial experiment was then designed to assess the influence and interactions of these variables over the following ranges; 150-210A current, 0-4 mm separation and 0.6-0.9m/min travel speed. This resulted in an experimental matrix of 18 welding conditions; the remaining parameters were as in the initial trials i.e. 60° torch angle, 5.2 kW laser power, -3 mm laser focus position, 1 mm diameter wire, 20 mm electrode extension and Ar-18% CO2-2% O2 shielding gas. Welds were assessed in terms of depth of penetration and weld flaw level (i.e. porosity and solidification cracking).
3. Results and discussion
3.1 General Observations
The initial trials showed that the two processes were successfully combined to give stable welding conditions using the set-up and conditions described in sections 2.3.1 and 2.3.2. The degree of spatter was similar to that obtained when using GMAW alone, but the resulting weld beads were wider and less convex than for the GMA process alone. A large, bright plasma was observed, similar to that for the GMA process.
Figure 6 shows macrosections taken from the initial trials of bead on plate welds to enable comparisons to be made between the two processes alone and hybrid welding. The depth of penetration was 1.6 mm for GMAW alone; 3.9mm (0mm focus) and 4.9mm (-3mm focus) for laser alone; 5.3mm (0mm focus) and 5.7mm (-3mm focus) for the hybrid process. Minor porosity was present but the projected area (summed in accordance with BS EN 4515:2000) was less than 1%.
Fig. 6. Macrosections of initial trial welds: 5.2kW laser power, 130A/26V GMAW setting, 0.6 m/min travel speed
a) Arc only
c) Hybrid
The hybrid process therefore increased the degree of penetration by about 20% over that for laser welding alone.
3.2 Weld Penetration
The influences of GMA current and arc-laser separation distance on depth of weld penetration at both travel speeds are shown in Figure 7 for the case of the GMA process leading and in Figure 8 for the laser process leading.
Fig. 7. Influence of welding parameters on weld penetration - GMA process leading
Fig. 8. Influence of welding parameters on weld penetration - Laser process leading
In general terms, an increase in the depth of penetration was associated with
- an increase in GMA current
- a decrease in welding speed
- zero arc-laser separation.
The results were in line with expectation and can be explained simply in terms of greater energy density giving rise to greater penetration. At a travel speed of 0.6m/min, the maximum penetration obtained was 7.5mm; at 0.9m/min the maximum penetration obtained was 6.5mm.
The results obtained at a travel speed of 0.6 m/min with the GMA process leading did not follow the above trends and the maximum penetration was associated with the minimum GMA current and zero arc-laser separation. These conditions reduced the effectiveness of the hybrid process and, as a large molten pool resulted, it is possible that molten pool interference with the laser keyhole may be responsible. It may, therefore, be necessary to restrict the molten pool size in order to maximise the benefits of hybrid welding. For commercial reasons, welding at a travel speed of 0.9 m/min is much more attractive than 0.6 m/min and further work will be carried out at the higher welding speeds.
In the present work, the influence of which process was leading was less significant than the other variables. When the laser is leading the GMA torch is 'pushing' and when welding with the GMA process above, 'pushing' normally results in reduced penetration. It is clear that further work is required to understand the detailed interactions in the weld process zone.
3.3 Joint Quality
All welds were radio graphed and no cracks or planar flaws were detected. There was, however, a degree of porosity and the results are summarised in Figure 9 for the GMA process leading and in Figure 10 for the laser process leading.
Fig. 9. Porosity level as a function of welding parameters - GMA process leading
Fig. 10. Porosity level as a function of welding
In general, porosity levels were low and satisfied the requirements of BS EN 4515: 2000. The maximum level of porosity found was approximately 0.8% in terms of projected area, which occurred at the slower welding speed of 0.6 m/min.At the higher speed, 0.9 m/min, porosity levels were below 0.4% projected area.
3.4 Weld Metal Microstructure
A typical weld metal microstructure is shown in Figure 11. The microstructure is predominantly acicular ferrite. No significant differences in microstructure were observed between the upper and lower sections of theweld.
Fig. 11. Hybrid weld metal microstructure, 0.6m/min travel speed, 5.2kW laser power, 210A GMA current
3.5 Joint Hardness
The results of hardness traverses across a hybrid weldment are summarised in Table 3. The maximum weld metal hardness was 280HV5 and the maximum HAZ value was 310HV5. As the proposed application is a non-sour environment these values are acceptable.
Table 3 Summary of hardness survey (hybrid laser-arc welding, GMA current 210A, arc-laser separation 0mm, travel speed 0.9 m/min.
Parent Material HV5 | Weld Metal HV5 | Heat Affected Zone HV5 |
 |  |  |
Results presented as | 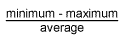 |
4. Continuing developments
The present work formed the initial stages of a continuing programme. Studies are continuing to define a robust welding procedure that will incorporate:
- higher laser powers (~9kW)
- optimisation of joint edge preparation
- all positional welding
- satisfying code requirements for joint quality and properties, in particular hardness and toughness
- grades of steel up to API 5L-X80
- achievement of significant economic advantage over current GMAW practice.
5. Concluding remarks
Based on a review of candidate welding processes for land-lay of pipelines and initial experimental trials it seems appropriate to draw the following conclusions:
- Hybrid Nd:YAG laser/gas metal arc welding offers opportunities for cost savings when laying new land pipelines.
- Stable welding conditions were established for the hybrid process which gave rise to an increase in penetration of approximately 20% over that for the laser welding alone.
- In the present work, the choice of leading process did not significantly influence the results and maximum penetration (7.5 mm) was achieved at a travel speed of 0.6 m/min, zero arc-laser gap, 5.2kW laser power and 210 A current.
- No cracks were observed and porosity levels were below 0.8% projected area.
- The maximum hardness (310 HV5) was found in the heat affected zone and is below the upper limit for non-sour conditions.
6. Acknowledgements
The majority of this work was funded by BP Exploration. P L Baines carried out the metallographic examinations.
7. References
- R Espiner 'BP's Pipeline Cost Reduction Project' Int. Conf. 'New Developments in Land Pipeline' Pipeline Industries Guild, December 2001.
- G Hutt 'Radial friction welding for offshore pipelines' Duplex stainless steels, 5th World Conference, 1997.
- C S Punshon et al 'Reduced pressure electron beam welding for steel pipelines' Proc. 6th Int. Colloq. Welding and Melting by Electron Beams, CISFFEL, Toulon, 15-19 June 1998.
- G S Booth and J Hammond 'Low cost welding techniques for pipelines', 'The Onshore Pipeline Cost Reduction Conference', Pipes and Pipelines International, April 2000.
- Gainaud et al 'Laser orbital welding applied to offshore pipeline construction' 3rd international conference on pipeline technology, May 2000.