Ceramics are an incredibly diverse family of materials whose members span traditional ceramics (such as pottery and refractories) to the modern day
engineering ceramics (such as alumina and silicon nitride) found in electronic devices, aerospace components and cutting tools.
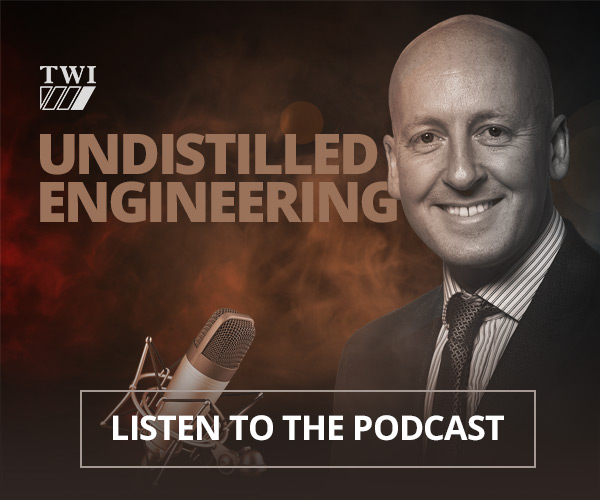
Whilst the most extravagant claims of the 1980s in favour of advanced ceramic materials (such as the all ceramic engine) have largely proved inaccurate, it is true to say that ceramics have established themselves as key engineering materials.
When used in conjunction with other materials, usually metals, they provide added functionality to components thereby improving application performance, once the appropriate joint design and technology have been identified.
Ceramic materials
Ceramics exhibit very strong ionic and/or covalent bonding (stronger than the metallic bond) and this confers the properties commonly associated with ceramics: high hardness, high compressive strength, low thermal and electrical conductivity and chemical inertness.
This strong bonding also accounts for the less attractive properties of ceramics, such as low ductility and low tensile strength. The wider range of properties, however, is not widely appreciated. For example, whilst ceramics are perceived as electrical and thermal insulators, ceramic oxides (initially based on Y-Ba-Cu-O) are the basis for high temperature superconductivity. Diamond, beryllia and silicon carbide have a higher thermal conductivity than aluminium or copper.
Control of the microstructure can overcome inherent stiffness to allow the production of ceramic springs, and ceramic composites have been produced with a fracture toughness about half that of steel.
The main compositional classes of engineering ceramics are the oxides, nitrides and carbides. The Table gives the general properties of the most used ceramics.
Table 1 Properties of ceramics
|
Ceramic | Melting point (°C) | Density (g/cm 3) | Strength (MPa) | Coefficient of thermal expansion (x 10 -6/°C) | Thermal conductivity (W/m.K) | Elastic Modulus (GPa) |
BeO |
2530 |
3.1 |
246 |
7.4 |
210 |
400 |
Al 2O 3 |
2050 |
4.0 |
455 |
8.0 |
40 |
380 |
ZrO 2 |
2700 |
5.6 |
175 |
10.5 |
19 |
140 |
AlN |
1900 |
3.3 |
441 |
4.4 |
180 |
320 |
Si 3N 4 |
1900 |
3.2 |
210 |
3.0 |
17 |
175 |
B 4C |
2350 |
2.5 |
350 |
4.3 |
25 |
450 |
SiC |
2700 |
3.2 |
140 |
4.3 |
50 |
210 |
WC |
2377 |
15.8 |
600 |
5.2 |
- |
700 |
Diamond |
3000 |
3.5 |
1500 |
0.5 |
2000 |
500 |
Oxides
Aluminium oxide (Al2O3) and zirconia (ZrO2) are the most commonly used engineering grade oxide ceramics, with alumina being the most used ceramic by far in terms of both tonnage and value.
Nitrides
Silicon nitride (Si3N4), and aluminium nitride (AlN) are the main advanced engineering ceramics in this category. There is a wide range of grades and types of these materials, particularly of silicon nitride with each grade having specific properties.
Carbides
Silicon carbide (SiC) is widely used for its high thermal conductivity, corrosion resistance and hardness, although as an engineering ceramic its toughness is lower than that of some silicon nitride grades. Boron carbide (B4C) is the third hardest industrial material (after diamond and cubic boron nitride) and is used for components needing very high wear performance.
Ceramic-based composites
Ceramics are used as the reinforcement of composite systems such as GRP (glass reinforced plastics) and metal matrix composites such as alumina reinforced aluminium (Al/Al 2O 3). Advanced ceramic materials are also used as the matrix materials in composites. Currently the most widely available materials are based on SiC and carbon.
Joining
There are many possible techniques for joining ceramics to themselves and to dissimilar materials. These technologies range from mechanical fixturing, such as with bolts and screw threads, to direct bonding. Fig.1 gives an overview of these methods.
The selection of one of these techniques to manufacture a particular component will depend on a number of factors including:
- desired component function eg strength, electrical insulation or wear resistance
- materials to be joined
- operational temperature
- applied stress
- required level of joint hermeticity
- component design
- cost
Whilst all these considerations must be taken into account, generally the two important factors are the similarity of the materials to be joined and the required temperature capability. Fig. 2 gives the temperature capability of a number of joining media.
When joining ceramics to metals it is necessary to create an interface between the materials. In general the interface must accommodate the following:
- the difference in coefficient of thermal expansion (CTE)
- bond type ie ionic/covalent for ceramics ranging to the metallic bond
- crystallographic lattice mismatch between the ceramic and metal
Applications
Compared to metals and plastics, ceramics are hard, non-combustible and inert. Thus they can be used in high temperature, corrosive and tribological applications. These applications rely on combinations of properties that are unique to industrial ceramics and which include:
- retention of properties at high temperature
- low coefficient of friction (particularly at high loads and low levels of lubrication)
- low coefficient of expansion
- corrosion resistance
- thermal insulation
- electrical insulation
- low density
Engineering ceramics are used to fabricate components for applications in many industrial sectors, including ceramic substrates for electronic devices (Fig. 3), turbocharger rotors (Fig. 4), and tappet heads for use in automotive engines. Other examples of where advanced ceramics are used include oil-free bearings in food processing equipment, aerospace turbine blades, nuclear fuel rods, lightweight armour, cutting tools, abrasives, thermal barriers and furnace/kiln furniture.
Summary
When selecting a material for use in a specific component the applicability and suitability of the candidate materials need to be considered in detail. When a ceramic material is being selected the fitness-for-purpose criteria that should be applied include:
- operational environment - atmosphere, temperature, applied stress, fatigue, exposure time
- predictable excursions beyond the usual, including mechanical impact or rapid heating/cooling
- design - ceramic materials are relatively intolerant of abrupt changes in cross-section such as notches, holes and corners
- joining - the role of the joint, its operational conditions and performance requirements and the joining techniques suitable for manufacture
- cost - as with all materials selection and component design questions, the cost and availability of the raw materials and all necessary fabrication techniques must be considered in the light of their suitability to provide a component with the required performance profile at a viable cost
Future development is likely to come from improved processing and fabrication techniques that will lower component costs or improve behaviour, an increasing demand for higher performance materials necessitating the use of more ceramics. Whilst it is difficult to predict new materials, improvements in existing ones can be readily foreseen. The most significant area of development is likely to be in the ceramic matrix composites.
Whilst existing composites based on SiC will improve as porosity levels are reduced by improved processing techniques, the development of high temperature oxide-based composites is likely to provide a competitor material system with wider applicability in the near future. In the future we can expect to see a still greater contribution to industrial growth and technological development from these materials.
This article was prepared by Alan Taylor.